I. What Processes are Involved in Muscle Contraction?
When you see gym rats or athletes lifting those heavy weights in the gym or training facility, it’s amazing to think that all of that strength when they flex those muscles all stems from cellular and subcellular processes — it’s definitely crazy to see that something so miniscule could help propel such a feat.
In regards to our articles talking about the musculoskeletal system, the actual physiological process of muscle contraction is a little more dense and complex due to the various players and pathways associated with muscle contraction; however, as we always emphasize, let’s take it step by step to make things a little bit easier to digest!
II. Physiological Processes Leading to Muscle Contraction
As mentioned above, it’s much easier to understand how muscle contraction works if we can break it down into smaller chunks and eventually bring them all back together to understand the whole concept.
We’ll break down muscle contraction into 2 parts: 1) activation of the neuromuscular junction and 2) cross bridge cycling! Additionally, note that the section covering activation of the neuromuscular junction is specific for the skeletal muscle only as this is what the MCAT focuses on!A. Activation of the Neuromuscular Junction
Recall that the neuromuscular junction is a specialized synapse composed of an efferent neuron and a muscle fiber/cell(s). In a nutshell, the neuromuscular junction is where the efferent neuron releases acetylcholine which binds to receptors on the muscle cell which initiates a transduction pathway leading to muscle contraction — let’s break it down step by step!
¹An efferent (exiting) motor signal from the CNS is sent and results in an action potential propagating down the axon of the efferent neuron. ²At the axon terminal, the action potential triggers the uptake of Ca⁺² ions via the opening of a transport protein.
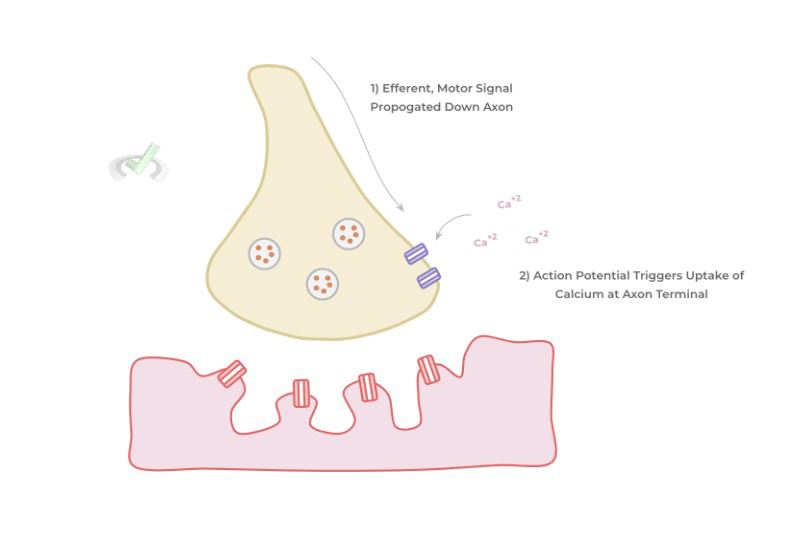
³Within the axon terminal are vesicles which contain the neurotransmitter acetylcholine. The uptake of calcium is the key trigger which initiates vesicle exocytosis, allowing for the release of acetylcholine across the synapse!
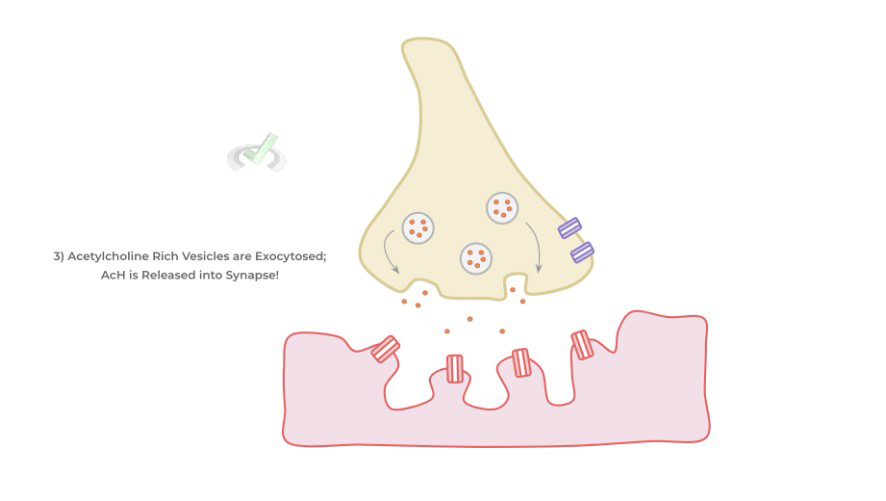
⁴After traversing across the synapse, acetylcholine then binds to nicotinic receptors located on the muscle cell membrane (which is called the sarcolemma). The receptors are ligand gated ion channels which means that after a molecule binds to them, an ion channel is open.
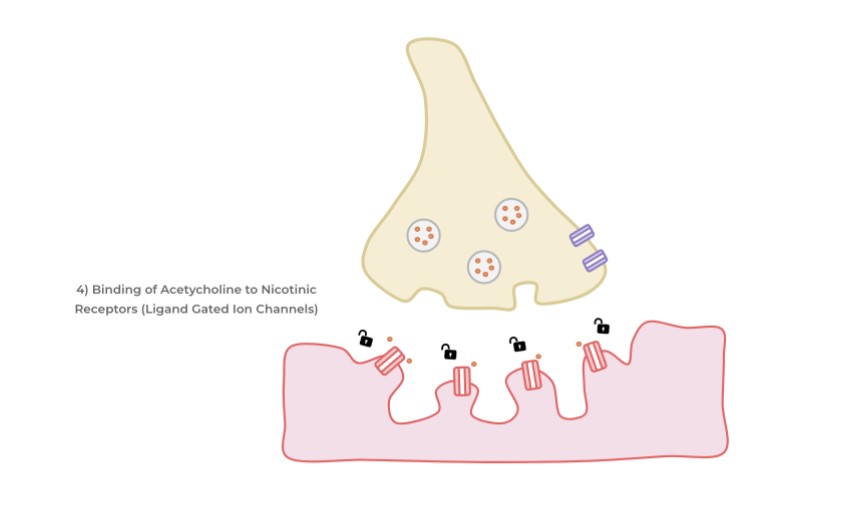
⁵The opening of the ligand gated ion channels allows for the influx of Na⁺ into the muscle cell resulting in depolarization and an eventual action potential in the muscle cell.
⁶The action potential is propagated from the sarcolemma towards the sarcoplasmic reticulum, which then results in the release of the stored Ca⁺² ions. These calcium ions will be crucial in the next phase of muscle contraction as we’ll get into in the next section!
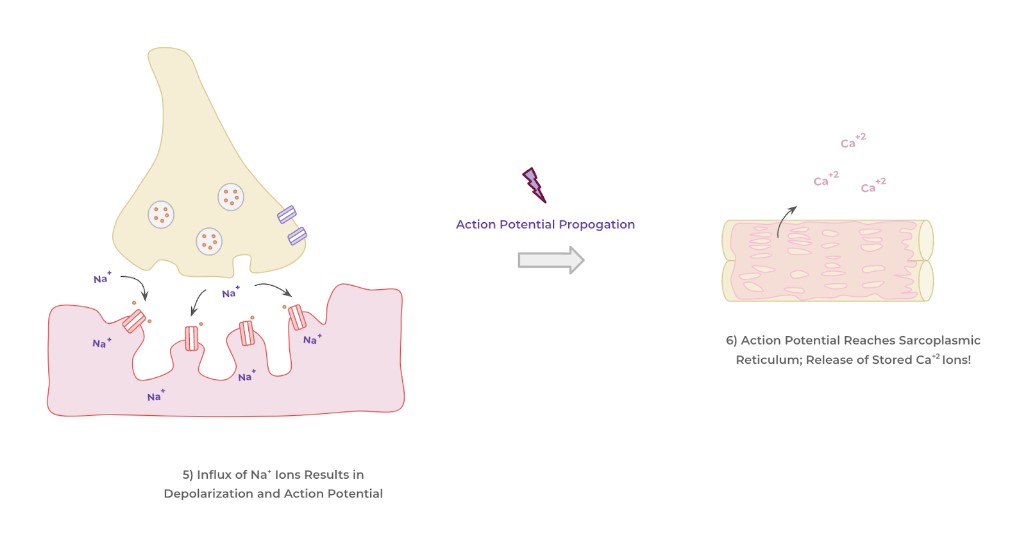
B. Sliding Filament Theory
Though this name sounds a little foreign, the sliding filament theory is essentially a possible explanation which postulates that muscle contraction occurs due to the binding and sliding of actin and myosin protein filaments with one another!
Cross bridge cycling is actually a smaller component of the overall theory and refers to molecular interactions that take place between the actin and myosin protein filaments which results in muscle contraction!
I. Importance of Troponin, Tropomyosin, and Calcium Ion Binding
An important concept regarding the sliding filament theory is the role of troponin and tropomyosin which are 2 proteins critical in regulating the binding of myosin and actin to prevent sustained muscle contraction.
When there’s no stimulus for contraction, tropomyosin is positioned in a way where the protein blocks the myosin binding sites of the actin filaments so that myosin CANNOT bind to actin and initiate contraction.
However, under stimulation for muscle contraction, Ca⁺² released from the sarcoplasmic reticulum can bind to troponin —- this initiates a conformational change in tropomyosin where it now exposes the myosin binding sites on the actin filament.
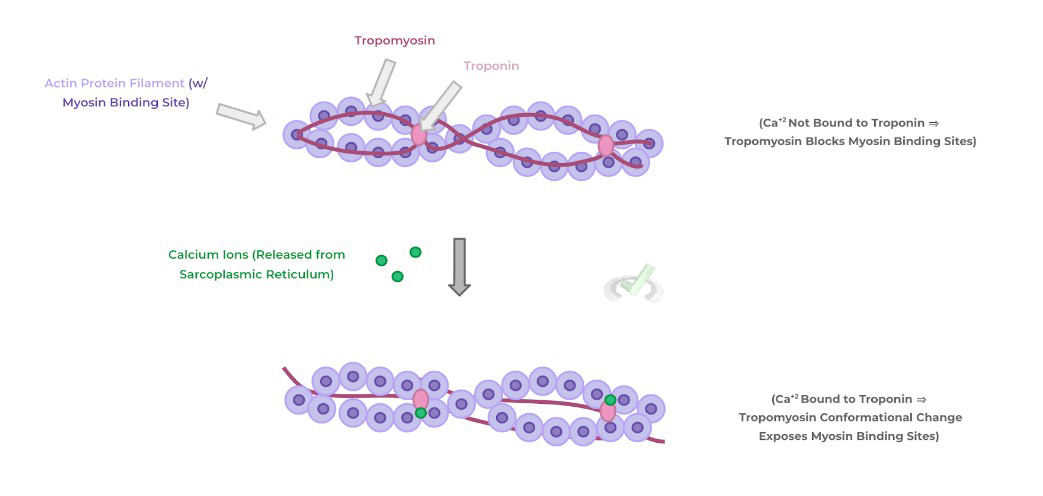
Myosin can now bind onto actin and initiate the process of cross bridge cycling which involves various changes in the conformational state of the myosin protein as we’ll see!
II. Cross Bridge Cycling
As mentioned above, cross bridge cycling is governed by how various substrates bound to the myosin globin head induces conformational changes!
¹When ADP, Pᵢ is bound to the globin head, the myosin protein is in a “cocked”, meaning it’s bound actin and has a high energy configuration in order to initiate a power stroke in the next step!
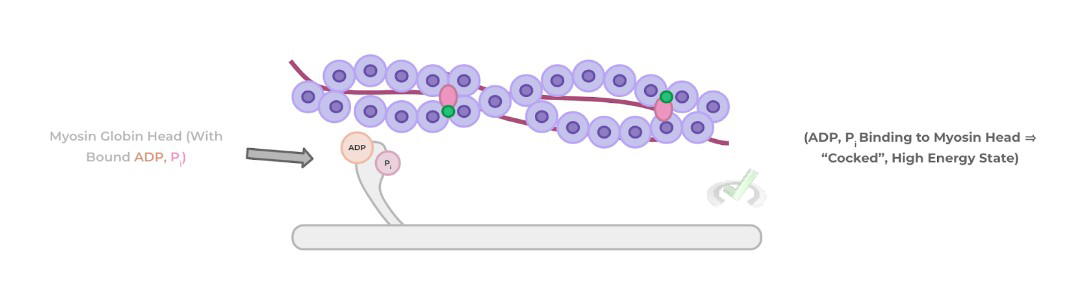
²Upon dissociation of ADP and Pᵢ, the myosin globin head initiates a power stroke which allows for the sliding of the actin filament! Note, that the energy that powers this stroke comes from the high energy configuration of the previous conformation of the myosin head.
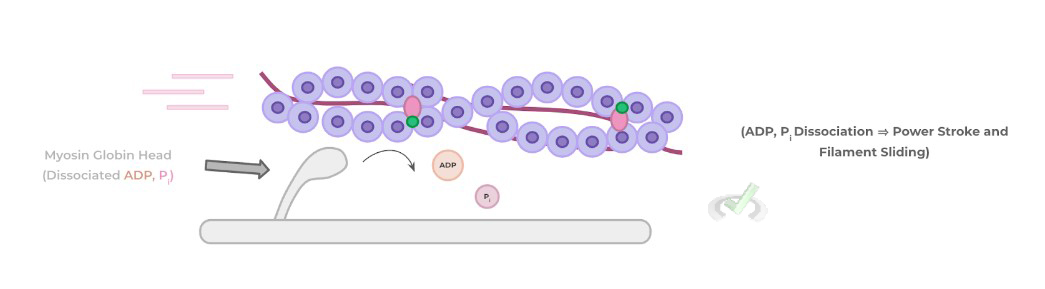
³The myosin head then binds a molecule of ATP — this binding of ATP triggers the dissociation of the myosin head from actin. It’s this conformational change which allows for muscle relaxation to occur after the power stroke.
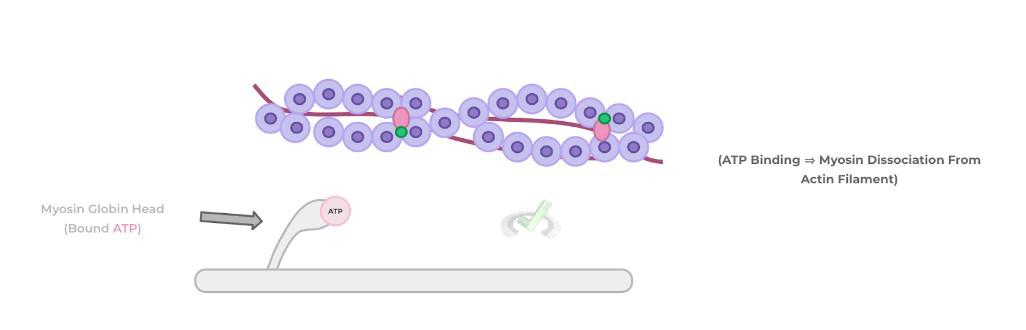
⁴Finally, the bound ATP undergoes hydrolysis and is broken down back to ADP, Pᵢ. Just like in the first conformation, this results in the binding again of myosin to the actin filament. Again, we have the myosin head loaded in a “cocked”, high energy state ready to repeat the cycle.
Sometimes, it’s easy to mistake that ATP hydrolysis is the process which results in the power stroke — remember, it’s the dissociation of ADP, Pᵢ which results in the power stroke! Take a look below at a summation of the process!
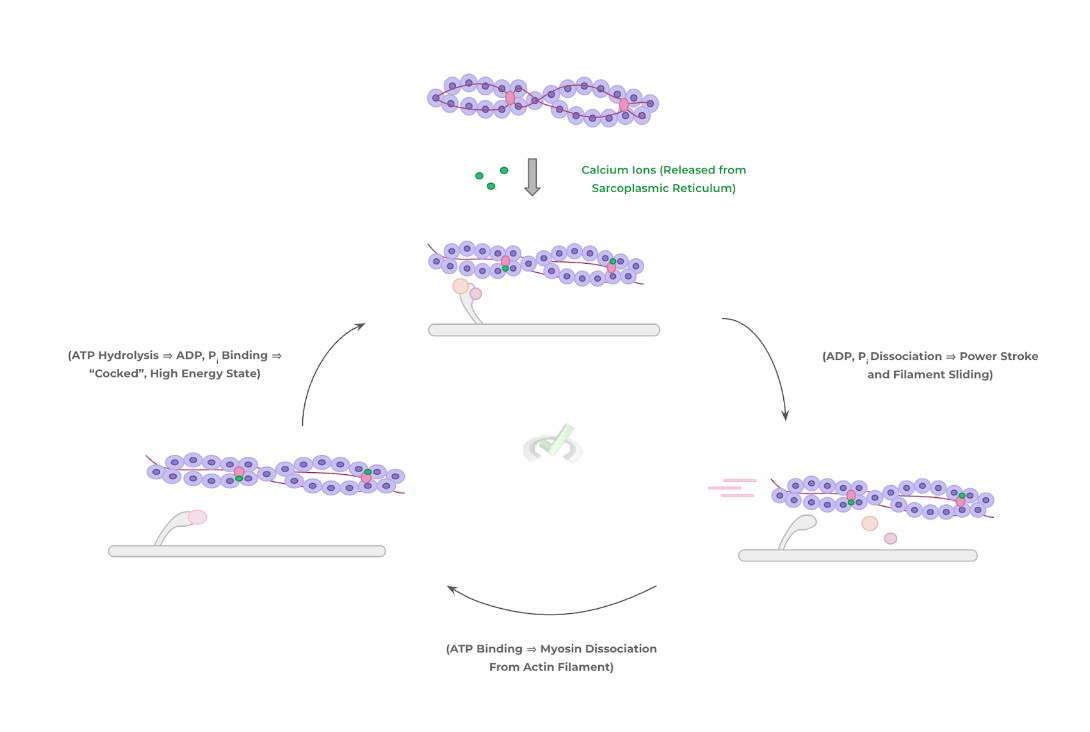
III. Bridge/Overlap
It’s important to note that there are actually 2 types of receptors: nicotinic and muscarinic receptors. As such, it’s easy to get confused between the 2 receptors especially where they’re located — let’s go ahead and give a brief breakdown of the receptors and key differences that help distinguish them!
I. Nicotinic v.s. Muscarinic Receptors
As mentioned above, nicotinic receptors are located on the sarcolemma (muscle cell membrane) and are associated with the neuromuscular junction! These are the receptors that acetylcholine will bind to in order to stimulate an action potential in the muscle fiber to initiate muscle contraction!
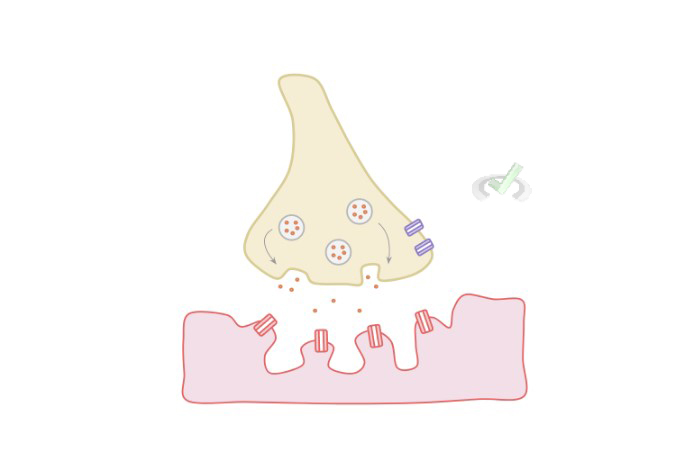
Conversely, muscarinic receptors are present on primarily autonomic ganglia as well as postganglionic target organs of the parasympathetic nervous system!
Recall that acetylcholine is the neurotransmitter released by preganglionic autonomic fibers (both in the sympathetic and parasympathetic divisions) and is released by post ganglionic parasympathetic fibers.
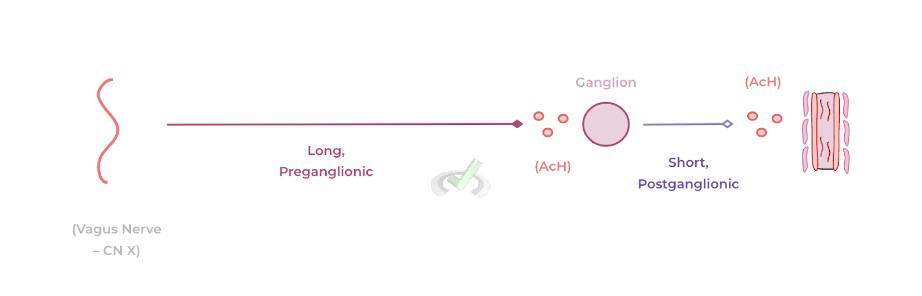
IV. Wrap Up/Key Terms
Let’s take this time to wrap up & concisely summarize what we covered above in the article!
A. Activation of the Neuromuscular Junction
The neuromuscular junction is a specialized synapse between an efferent motor neuron and a muscle cell(s) which when activated initiates muscle contraction. After the propagation of an action potential down the efferent neuron, there’s an increased influx of Ca⁺² ions into the axon terminal.
The increased influx of Ca⁺² ion is a stimulus for the exocytosis of vesicles containing acetylcholine which are then released into the synaptic cleft and bind to nicotinic receptors located on the sarcolemma.
Nicotinic receptors are actually ligand gated ion channels which means upon binding of acetylcholine, an ion channel for Na⁺ opens and allows for its influx. This triggers an action potential in the myofiber which is then propagated to the sarcoplasmic reticulum allowing for the release of stored Ca⁺² ions.
B. Sliding Filament Theory
This theory postulates that the muscle contraction occurs due to the interactions and sliding of actin and myosin filaments. On a more specific scale, the process of cross bridge cycling refers to the actual molecular interactions and conformation changes which allow for muscle contraction to occur.
I. Importance of Troponin, Tropomyosin, and Calcium Ion Binding
Troponin and tropomyosin are important proteins which interact with actin to prevent sustained muscle contraction. Under no stimulation, tropomyosin covers the myosin binding sites on the actin filaments.
Upon stimulation of muscle contraction, the Ca+2 ions released by the sarcoplasmic reticulum bind onto troponin which causes a conformational change to tropomyosin allowing it to expose the myosin binding sites!
II. Cross Bridge Cycling
This process involves changes in the conformational state of the myosin globin head based on what substrates are bound to it!
When ADP, Pᵢ is bound, the myosin head is in a “cocked”, high energy state bound to actin. Upon dissociation, the myosin head initiates a power stroke which allows for the sliding of the filaments and ultimately results in muscle contraction!
The binding of ATP to the myosin head allows for myosin dissociation from actin which results in muscle relaxation. Finally, ATP is hydrolyzed back to ADP, Pᵢ and another cycle can repeat!
V. Practice
Take a look at these practice questions to see and solidify your understanding!
Sample Practice Question 1
Myasthenia Gravis is an autoimmune condition characterized by the formation of autoantibodies which attack and destroy the nicotinic receptors of the neuromuscular junction. Which of the following physiological effects would be a ramification of this disease?
A. Muscle Spasm (Increased Muscle Contraction)
B. Muscle Weakness (Decreased Muscle Contraction)
C. Increased Cross Bridge Cycling
D. Increased Myofiber Depolarization
Answer: B. Muscle Weakness (Decreased Muscle Contraction)
Because the nicotinic receptors on the sarcolemma are destroyed in this disease, the activation of the myofiber for muscle contraction is decreased because there’d be decreased binding from the released acetylcholine with the nicotinic receptors
Sample Practice Question 2
Rigor mortis is characterized by increased stiffness of muscle in dead people due to the inability of the muscle to relax. Which of the following substrates which binds to the myosin head is most likely DECREASED and leads to this condition?
A. ADP, Pᵢ
B. ATP
C. Ca⁺² Ions
D. Acetylcholine
Answer: B. ATP
Recall that the binding of ATP to the myosin head results in dissociation of the myosin head with actin allowing for muscle relaxation. As such, a decreased amount of ATP would result in the inability of myosin to detach from actin resulting in decreased muscle relaxation.
Additionally, note that Ca+2 and acetylcholine do not bind to the myosin globin head but rather bind to troponin and nicotinic receptors, respectively.